Thousands of bubbles to visualize the brain
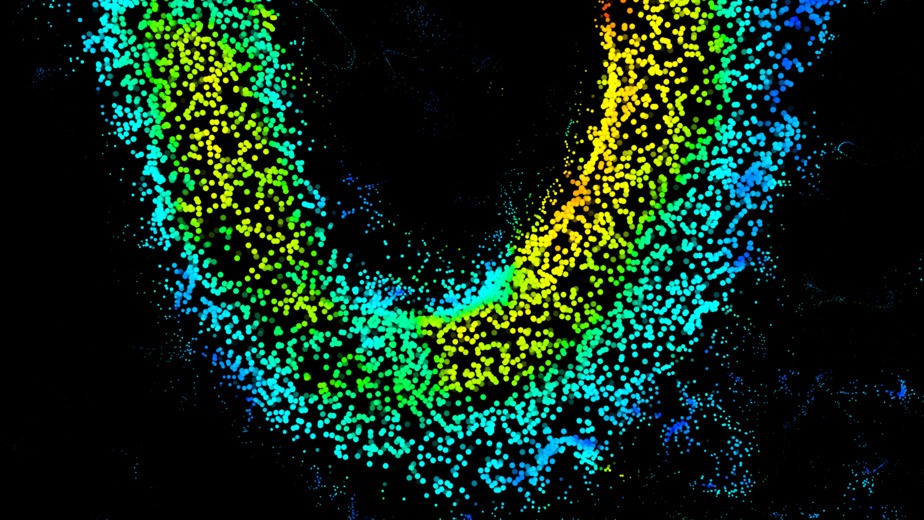
IMAGE PROVIDED BY THE PHYSICAL LABORATORY FOR MEDICINE AT ESPCI PARIS
The bubbles are followed one by one by ultrasound imaging, to reconstruct a very detailed map of blood flow in the brain. The colors vary according to the speed of the blood: dark blue corresponds to very slow speeds (close to 0 mm / s), green and yellow to intermediate speeds (close to 100-150 mm / s), and red at fast speeds (close to 250 mm / s). As in a river, we notice on the left that the “current” is stronger in the center than on the edge of the vessel.
This technique is based on an injection of very small bubbles, already used today by doctors to better see the blood in cardiac ultrasound. Carried away by the blood flow, these bubbles roam the whole body for about ten minutes. Meanwhile, an ultrasound machine records thousands of images of the brain per second, much like a camera would take a series of shots in a row. Powerful calculations are then launched to locate and follow each bubble from image to image, along the blood vessels. At the end of the day, all of these bubbles added together make up an extremely detailed map of blood flow in the brain. This method using ultrasound makes it possible to locate the bubbles, to visualize the blood vessels on a microscopic scale: hence its name, ultrasound localization microscopy.
See better, understand better, diagnose better
À partir de ce type de carte, il sera peut-être possible de détecter des écoulements anormaux du sang, et de les relier au développement de certaines maladies cérébrales. « C’est un peu comme inspecter la plomberie du cerveau à de nouvelles échelles : on va pouvoir repérer des problèmes de malformations […], problems related to cerebrovascular accidents (CVA)… ”, details Charlie Demené, lecturer and researcher at the Physics for Medicine laboratory at ESPCI Paris.
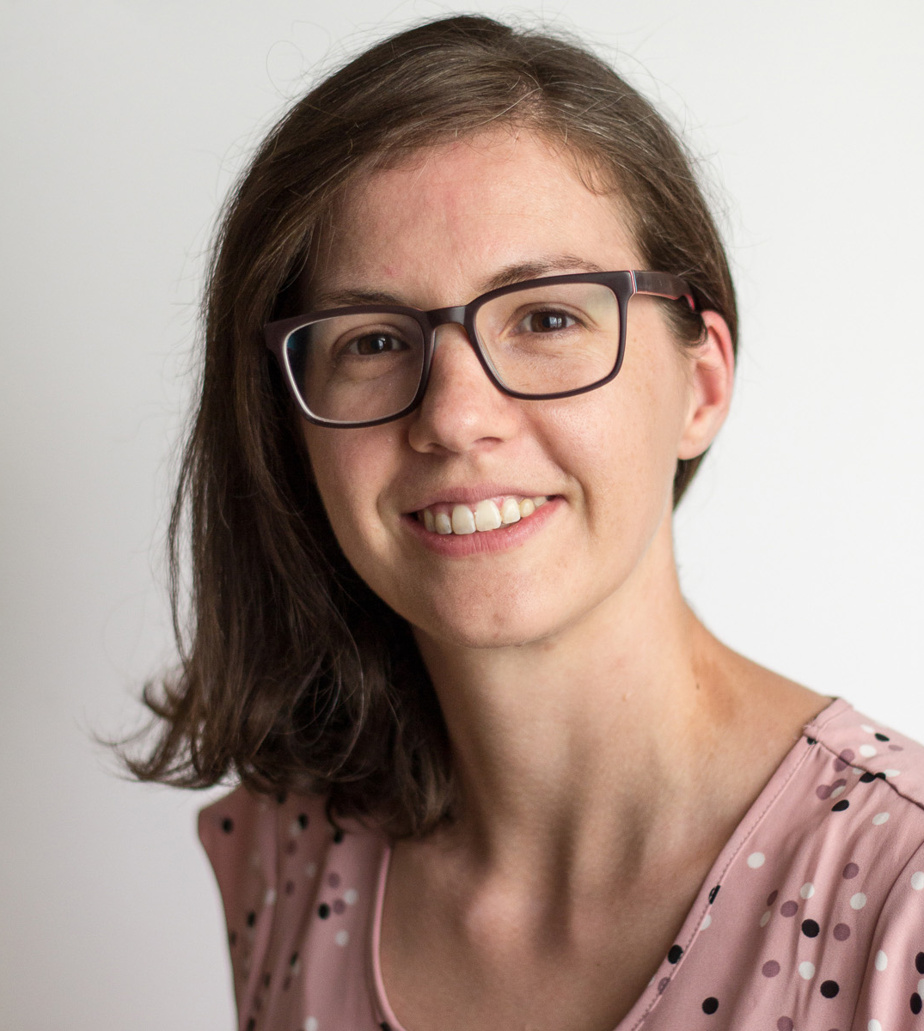
PHOTO DAVID KILLING, SUPPLIED BY MEAGHAN O’REILLY
Meaghan O’Reilly, researcher at the Sunnybrook Health Sciences Center at the University of Toronto
There is enormous potential here to better understand diseases, to better diagnose them, but also to identify new mechanisms and acquire new knowledge in our understanding of the brain.
Meaghan O’Reilly, researcher at the Sunnybrook Health Sciences Center at the University of Toronto
A first in humans
As with a standard ultrasound, this method does not require surgery or radiation, and is both portable and inexpensive. It is also very recent: the bubble localization method is inspired by a high-resolution fluorescence microscopy technique, developed in the early 2000s by Eric Betzig, Stefan W. Hell and William E. Moerner. The latter were rewarded for their work with the Nobel Prize in chemistry in 2014. The application of this method to ultrasound was presented in an article by Nature one year later, in 2015. She has since aroused a great deal of interest in the field of ultrasound imaging research. For the first time, results were obtained in three humans and published in an article published in March 2021 in Nature Biomedical Engineering, of which Charlie Demené is the first author.
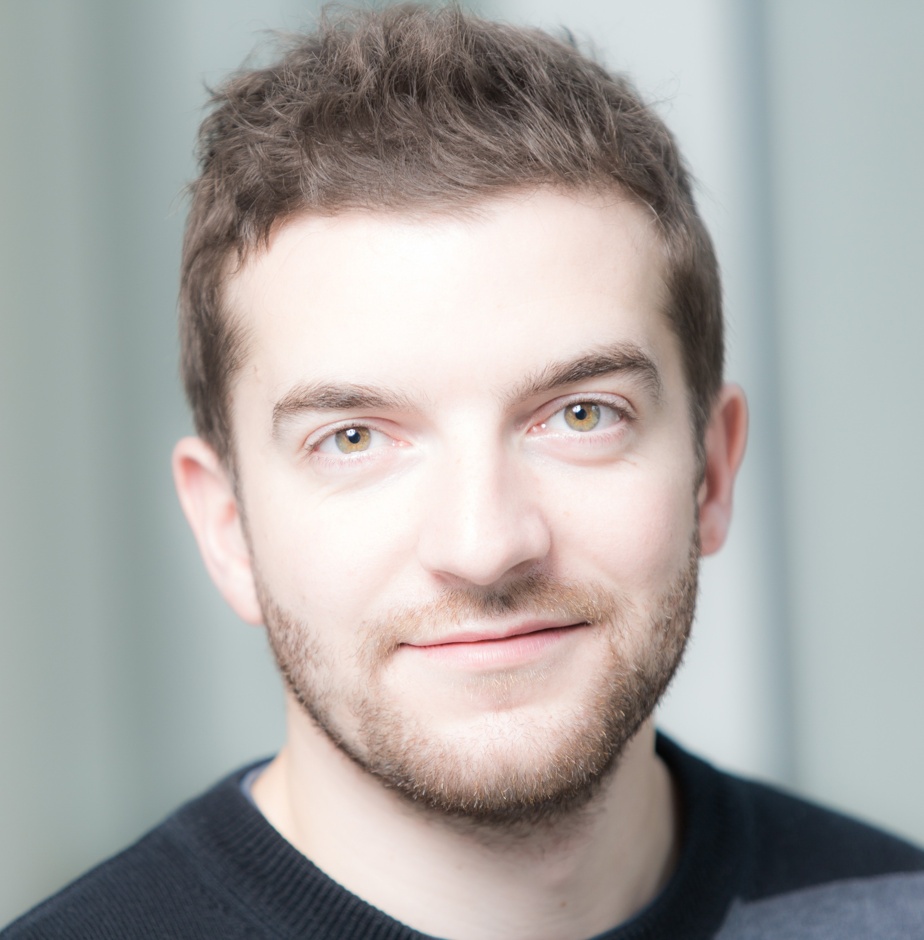
PHOTO BENJAMIN BOCCAS, PROVIDED BY CHARLIE DEMENÉ
Charlie Demené, lecturer and researcher at the Physics for Medicine laboratory at ESPCI Paris
Applying this method to humans is a real tour de force, according to Meaghan O’Reilly, a researcher at the Sunnybrook Health Sciences Center at the University of Toronto and co-author of an article on ultrasound localization microscopy. Ultrasound imaging is based on the ability of ultrasound to pass through tissues (vessels, muscles, fat, etc.) in order to make an image of it. But the bones remain a difficult barrier to cross: “Doing this through the human skull is not trivial. The skull attenuates and distorts the ultrasound as it passes through it, ”she explains.
Some challenges remain
Before seeing this method used daily by doctors, “some challenges still need to be met,” adds Meaghan O’Reilly.
Due to the thickness of the skull, images were captured where the bone is thinnest, i.e. through the temple. “We only have access to part of the brain,” Charlie Demené explains. “There is still a lot to do to get through thicker bone, and have more direct access to the brain. He also indicates in his article that in the long term, this method could make it possible to generate a very detailed 3D image of the entire brain, and thus open up new perspectives.
Image processing time could also be optimized. To build a very detailed map, the bubbles must have had time to travel through all the blood vessels, especially the finer ones: the longer you wait and take a large number of images, the more detailed the final map will be. Now, “for every second [d’images enregistrées], it takes almost three minutes of treatment, ”says Meaghan O’Reilly. For images taken for 45 seconds, as in the article, therefore, a doctor would have to wait more than two hours before getting a detailed map of the patient’s brain.
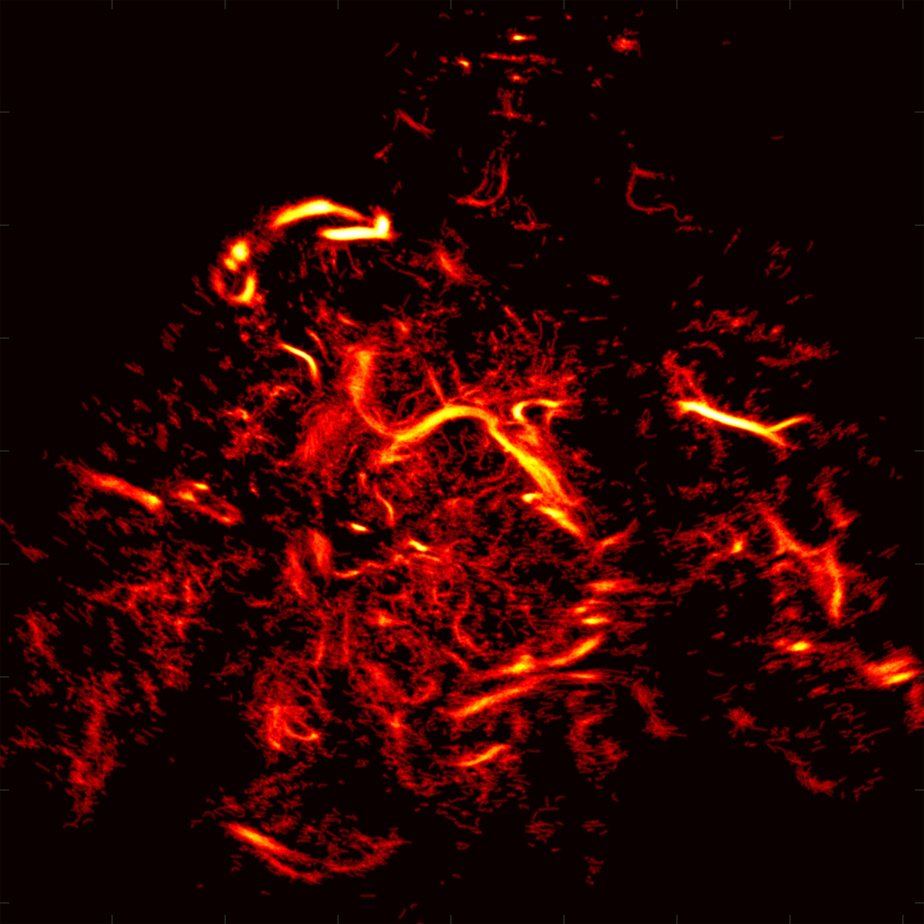
IMAGE PROVIDED BY THE PHYSICAL LABORATORY FOR MEDICINE AT ESPCI PARIS
Image of blood flow in the brain produced by Charlie Demené’s team: we see both large vessels (in yellow, in the center), but also thinner vessels (in red). The image is approximately 10 cm wide. The colors vary from yellow to red, depending on the number of bubbles that have passed through the vessel.
This method would facilitate the diagnosis of patients with cerebral diseases, but it would also be necessary to find treatments to take care of these people. For example, in the case of Alzheimer’s disease, “there are things that happen at the level of the microvascularization. [c’est-à-dire au niveau des petits vaisseaux sanguins dans le cerveau], in connection with the development of this disease, ”emphasizes Charlie Demené. But no treatment has yet been found to cure or slow its progression. “In the world of imaging, it’s complicated because we only work on the diagnostic side, we do not work on the curative side. […] We are working on our own brick, and we have to see ourselves as part of the collective, ”he says. “By taking small steps one after the other, we will be able to take better care of patients. “
In numbers
1 micrometer = 0.001 millimeter 40-100 micrometers: typical diameter of a hair
2.5 micrometers: average size of the bubbles used
25 micrometers: resolution achieved in humans with ultrasound localization microscopy
200 micrometers: typical resolution achieved in humans with conventional ultrasound imaging
Chloé Bourquin, author of the article and trainee journalist at Press, did a doctorate in biomedical engineering at Polytechnique Montréal in Jean Provost’s ultrasound laboratory. In her research, she uses a variant of ultrasound localization microscopy, to observe the pulsation of blood during the cardiac cycle in small blood vessels. Ultimately, this could help detect the early signs of neurodegenerative diseases such as dementia.